In 2010, it was discovered that “non-catalytic” proteins described in 2005 to promote the hydrolysis of crystalline chitin by chitinases are redox enzymes that use oxidative chemistry to attack glycosidic bonds in the most recalcitrant (i.e., crystalline) parts of insoluble polysaccharides such as cellulose and chitin. Today, these enzymes are generally referred to as Lytic Polysaccharide Monooxygenases or LPMOs.
LPMOs are unique in that they are small (typically less than 200 amino acids) and easy to produce, while at the same time being very powerful catalysts capable of selectively oxidizing non-activated C-H bonds. Notably, most LPMOs are interfacial enzymes that act on the surfaces of insoluble substrates, although additional activities, including activities on soluble oligosaccharides have also been described. Some LPMOs carry a carbohydrate-binding module (CBM) that increases substrate affinity.
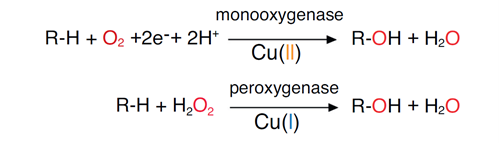
LPMOs are mono-copper enzymes that require electrons and an oxygen co-substrate for catalysis. Originally, these enzymes were thought to use molecular oxygen, hence “monooxygenase”, but it has later been shown that LPMOs also may carry out peroxygenase reactions, i.e. using H2O2, and that the latter reactions are much more efficient. While the monooxygenase reaction may not be so important in the reaction with a polysaccharide substrate, it is clear that LPMOs can activate molecular oxygen. It is worth noting that the catalytic copper site of LPMOs resembles a copper site in particulate methane monooxygenase (pMMO) that, until recently, was generally considered to be pMMO’s catalytic center.
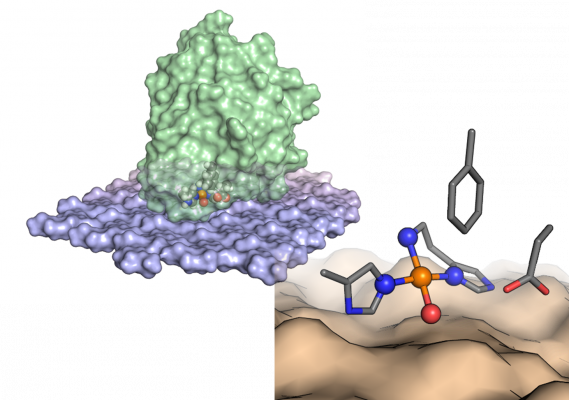
Despite huge interest in these enzymes, due to their intriguing catalytic power and industrial applications, many questions regarding their functionality remain unanswered. It can be argued that, so far, the potential of natural LPMOs has not been fully harnessed, nor has the potential to use LPMOs to catalyze other difficult reactions, next to polysaccharide oxidation.
While the copper site of LPMOs is highly conserved, sequence variation among natural LPMOs is huge. This variation includes the extended substrate-binding surface and, to a lesser extent, the second copper coordination shell. The surfaces of LPMOs seem highly evolvable, which gives opportunities to engineer these surfaces to bind to, and potentially act on, other polymeric substrates, such as plastics. Second shell variations may affect the redox properties of the copper site, potentially providing lessons for copper catalyst design.
Active site confinement is crucial for LPMO functionality and stability. In natural LPMOs, such confinement is provided by the substrate itself, which offers a surface for the LPMO to dock upon, shielding the exposed copper site from solution. Considering the evolvability of LPMO surfaces and the existence of natural LPMOs with “rugged” surfaces, we will explore the possibility to engineer binding pockets near the copper site that would allow the enzyme to selectively activate C-H bonds of smaller substrates, such as alkanes.
In CuBE, we will unravel how LPMOs work, how LPMOs can be made more efficient, and how we can make these enzymes catalyze new reactions.
For more information check these articles:
Vaaje-Kolstad, G.; Westereng, B.; Horn, S.J.; Liu, Z.; Zhai, H.; Sørlie, M.; Eijsink, V.G.; An oxidative enzyme boosting the enzymatic conversion of recalcitrant polysaccharides.. Science 2010, 330(6001), 219-22.
Quinlan, R.J.; Sweeney, M.D.; Lo Leggio, L.; Otten, H.; Poulsen, J.C.; Johansen, K.S.; Krogh, K.B.; Jørgensen, C.I.; Tovborg, M.; Anthonsen, A.; Tryfona, T.; Walter, C.P.; Dupree, P.; Xu, F.; Davies, G.J; Walton, P.H.; Insights into the oxidative degradation of cellulose by a copper metalloenzyme that exploits biomass components.. Proc Natl Acad Sci U S A. 2011, 108(37), 5079-84.
Kjaergaard, C.H.; Qayyum, M.F.; Wong, S.D.; Xu, F.; Hemsworth, G.R.; Walton, D.J.; Young, N.A.; Davies, G.J.; Walton, P.H.; Johansen, K.S.; Hodgson, K.O.; Hedman, B.; Solomon, EI.; Spectroscopic and computational insight into the activation of O2 by the mononuclear Cu center in polysaccharide monooxygenases. Proc Natl Acad Sci U S A., 2014, 111(24), 8797-802.
Kracher, D.; Scheiblbrandner, S.; Felice, A.K.; Breslmayr, E.; Preims, M.; Ludwicka, K.; Haltrich, D.; Eijsink, V.G.; Ludwig, R.; Extracellular electron transfer systems fuel cellulose oxidative degradation. Science. 2016, 352(6289):1098-101.
Kjaergaard, C.H.; Qayyum, M.F.; Wong, S.D.; Xu, F.; Hemsworth, G.R.; Walton, D.J.; Young, N.A.; Davies, G.J.; Walton, P.H.; Johansen, K.S.; Hodgson, K.O.; Hedman, B.; Solomon, EI.; The molecular basis of polysaccharide cleavage by lytic polysaccharide monooxygenases. Nat Chem Biol. 2016, 12(4):298-303.
Bissaro, B.; Røh,r A.K.; Skaugen, M.; Forsberg, Z.; Horn, S.J.; Vaaje-Kolstad, G.; Eijsink, V.G.H.; Fenton-type chemistry by a copper enzyme: molecular mechanism of polysaccharide oxidative cleavage.. BioRxiv 097022. 2016.
Bissaro, B.; Røhr, Å.K.; Müller, G.; Chylenski, P.; Skaugen, M.; Forsberg, Z.; Horn, S.J.; Vaaje-Kolstad, G.; Eijsink, V.G.H.; Oxidative cleavage of polysaccharides by monocopper enzymes depends on H2O2.. Nat Chem Biol. 2017, 13(10):1123-1128.
Kuusk, S.; Bissaro, B.; Kuusk, P.; Forsberg, Z.; Eijsink, V.G.H.; Sørlie, M.; Väljamäe, P.; Kinetics of H2O2-driven degradation of chitin by a bacterial lytic polysaccharide monooxygenase. J Biol Chem. 2018, 293(2):523-531.
Piotr Chylenski; Bastien Bissaro; Morten Sørlie; Åsmund K. Røhr; Anikó Várnai; Svein, J. Horn; Eijsink, V.G.H.; Lytic Polysaccharide Monooxygenases in Enzymatic Processing of Lignocellulosic Biomass. ACS Catalysis. 2019, 9, 4970-14991.
Bissaro, B.; Streit, B.; Isaksen, I.; Eijsink, V.G.H.; Beckham, G.T.; DuBois, J.L.; Røhr Å.K.; Molecular mechanism of the chitinolytic peroxygenase reaction. Proc Natl Acad Sci U S A. 2020, 117(3):1504-1513.
Jones, S.M.; Transue, W.J.; Meier, K.K.; Kelemen, B.; Solomon, E.I.; Kinetic analysis of amino acid radicals formed in H2O2-driven CuI LPMO reoxidation implicates dominant homolytic reactivity. Proc Natl Acad Sci U S A. 2020, 117 (22) 11916-11922.
Courtade, G.; Ciano, L.; Paradisi, A.; Lindley, P.J.; Forsberg, Z.; Sørlie, M.; Wimmer, R.; Davies, G.J.; Eijsink, V.G.H.; Walton, P.H.; Aachmann, F.L.; Mechanistic basis of substrate-O2 coupling within a chitin-active lytic polysaccharide monooxygenase: An integrated NMR/EPR study. Proc Natl Acad Sci U S A. 2020, 117(32):19178-19189.
Bissaro, B.; Kommedal, E.; Røhr, Å.K.; Eijsink, V.G.H.; Controlled depolymerization of cellulose by light-driven lytic polysaccharide oxygenases. Nat Commun. 2020, 11(1):890.
Stepnov A.A.; Forsberg, Z.; Sørlie, M.; Nguyen, G.S.; Wentzel, A.; Røhr, Å.K.; Eijsink, V.G.H.; Unraveling the roles of the reductant and free copper ions in LPMO kinetics. Biotechnol Biofuels. 2021, 14(1):28.